Steel or Polymeric? What's best for soil reinforcement!
- Michael Sorensen
- Jul 23, 2024
- 4 min read
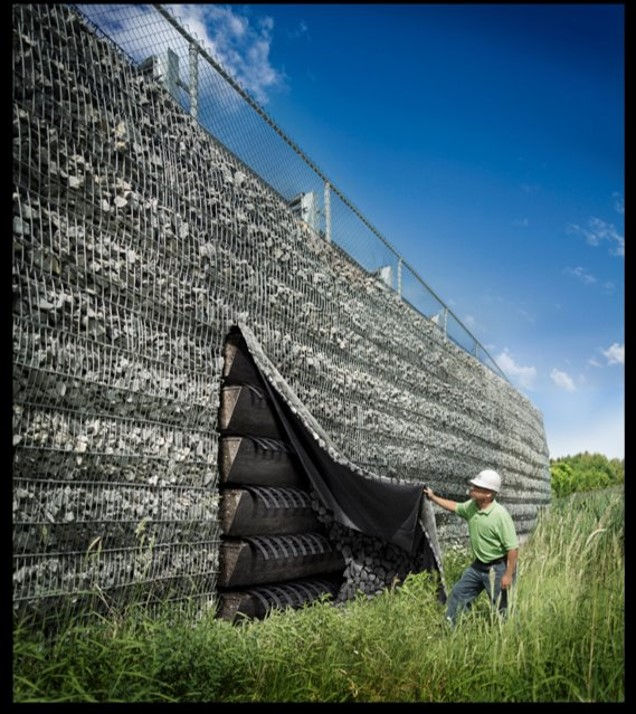
As a design engineer often faced with the complexities of bridge abutments and mechanically stabilized earth (MSE) walls, We may frequently ponder the optimal type of reinforcement to use. Terms like "extensible" and "inextensible" reinforcement can be confusing. They conjure images of stretchy, rubbery materials versus strong, rigid steel, but is this distinction accurate?
Defining Extensible and Inextensible Reinforcement
One of the major issues with these terms is the lack of clear definitions within our industry. The guidance documents we rely on, such as those from AASHTO, classify reinforcement as either extensible or inextensible without providing exact definitions. The New Zealand bridge manual offers a bit more insight, suggesting that inextensible reinforcement is usually steel, while extensible reinforcement is typically geogrid. However, it also mandates inextensible (steel) reinforcement in certain applications, adding to the confusion.
This brings up a common misconception: the belief that steel, being inherently stronger and stiffer than plastic, is automatically the superior choice for all applications. But is this always the case?
The Role of Steel and Geogrid Reinforcement
It's important to recognize that both steel and geogrid have their places in reinforcement. The key question is: can we define these terms more precisely, clarify what is required, and address the perceptions they carry?
Steel reinforcement is undeniably stiff. When compared with typical strain rates of engineering soils, it is practically inextensible. However, this stiffness can be a drawback in MSE wall design, as it may prevent the soil from fully mobilizing its strength. This is a critical consideration in design. Conversely, geogrids can be selected to be as stiff as needed, especially with advancements in high tenacity polyesters, polyvinyl alcohol grids, and even polyaramids.
Mobilizing Soil Strength and Reinforcement Strength
There is a fundamental difference in how reinforcement interacts with soil between truly inextensible elements and those that strain slightly. Soil is a frictional material with inherent strength, which we use in stability calculations. A small strain in the reinforcement at a critical point allows the soil to mobilize and the reinforcement to distribute load to the surrounding soil.
A recent FHWA publication states: “In reality, inextensible reinforcement restrains the formation of an active wedge. Since the soil does not contribute its full strength, the reinforcement must carry higher loads than extensible reinforcement to satisfy equilibrium. This could lead to the potential rupture of metallic reinforcement after exceeding its yield strength, requiring careful evaluation when considering inextensible reinforcement in LE analysis.”
Strain Shedding Between Layers of Reinforcement
Geosynthetic reinforcement, which strains slightly, allows the soil to mobilize and contribute to overall stability. This results in load shedding, where a given load can be redistributed to multiple layers of reinforcement. Geosynthetics can be managed to match the strain levels of the soil, unlike a truly rigid element.
Achieving Required Strength at Very Low Strain
While strength is crucial, the strain of reinforcement is also critical in some applications. In walls with rigid facings or reinforced soil structures supporting bridges or buildings, deformation can be detrimental. This is why authorities often insist on steel reinforcement.
However, geosynthetic reinforcement, while capable of straining, can be engineered to limit this strain to within required tolerances. Isochronous creep curves, which show strength versus strain over time, allow us to select appropriate products and grades. Typically, this results in strain levels around 1-2%, allowing some soil strength to mobilize while minimizing deformation.
It’s also important to note that any strain in the reinforcement only affects the stressed section, not the full length. For instance, a 6m length of geogrid designed to strain no more than 2% will not move 120mm. Only the portion intercepting the critical plane and the resultant anchor lengths experience maximum load, transferring it to the surrounding soil.
Degradation of the Reinforcement
For bridge abutments, reinforcement must maintain its design strength for over 100 years. Geosynthetic reinforcement faces degradation mechanisms such as creep rupture, installation damage, and environmental factors like hydrolysis for PET grids and oxidation for HDPE grids. These mechanisms are well understood and can be accounted for in design.
Similarly, steel reinforcement must consider corrosion and the impact of installation damage on corrosion coatings. Both types of reinforcement can meet long-term performance requirements, though site-specific conditions may make one more suitable than the other.
Conclusion
Geosynthetic reinforcement has a proven track record in bridge abutments, even in high seismic areas. The Japanese Rail's use of geosynthetic reinforced walls with rigid facings for high-speed trains is a testament to their durability and performance. Given this history, it may be time to consider strain-limited geosynthetic designs for bridge abutments, allowing for true value engineering as we continue to push the boundaries of research and experience.
By clearly defining and understanding the terms extensible and inextensible, we can make more informed decisions in our designs, leveraging the strengths of both steel and geogrid reinforcement.
Comments